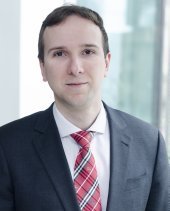
Alexandre Boutet, MD, PhD
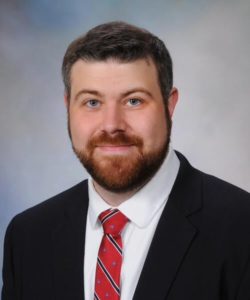
Erik H. Middlebrooks, MD
Alexandre Boutet / Erik H. Middlebrooks
On Wednesday, November 30th, at noon ET / 6PM CET, we hosted Alexandre Boutet and Erik H. Middlebrooks.
Alexandre Boutet, MD, PhD, is a neuroradiology fellow at the University of Toronto, and told us about “How to become an integral part of the functional neurosurgery team as a radiologist”.
Erik H. Middlebrooks, MD, is a Professor and Consultant of Neuroradiology and Neurosurgery and the Neuroradiology Program Director at Mayo Clinic. Beside his scientific presentation about “Bridging the Gap from Research to Clinical Decision Making in Epilepsy Neuromodulation”, he also gave us a glimpse at the “Person behind the science”.
Watch the recording with our interactive transcripts below!
so much for joining. Special thanks also to our speakers for taking the time to be here today and to my co-organizers, Gerard and Patricia, and also to Andy and our partners for the generous support in this event series. Today we're excited to hear from Alexandre Routet and Eric Milbrooks, and Alexandra is a Neuro-Radiology Fellow in Postdoc and Analyst at the University of Toronto. Eric is a professor of radiology, a consultant of neuroradiology and Neurosurgery and the Neuroradiology Program Director at Mayo Clinic in Florida. And besides Eric's scientific presentation, he will also give us a glimpse at the person behind the science. And then after the two talks, we will have some time for a shared discussion, which will involve both speakers. And here, we invite everyone to either raise your hands to ask a question live within the session, or you can also type questions into the chat. And obviously a sinus tide, unfortunately, we will try to prioritize questions that have received most upvotes from other attendees. But now without further ado, please join me in welcoming our first speaker, Alexandre Bouty to the virtual stage. Hi. So, first of all, thank you for doing this and having me. I was a little surprised when I got the invite, but I will try to share as much wisdom as as possible from my trainee perspective here today. So let me share my screen. Okay.
So I thought today I would do, just one second. I would do something maybe a bit more unexpected in terms of content since this is a brain stimulation talk and I'm sure we're all interested in stimulation and we've heard a lot about it, but I thought I would give maybe a slightly different perspective or on the field from a radiology point of view. So I titled the short speech today is how to become an integral part of the functional neurosurgery team as a radiologist. So first of all, I had to find a definition of what is a radiologist since I don't think it's clear for most people that are less familiar with the medical field. So that the official definition as per the American College of Radiology is that radiologists are medical doctors that specialize in diagnosing and treating injuries and diseases using medical imaging procedures, X-ray, CT, MRI, and nuclear medicine. So in a North American system, the path to becoming a radiologist is you first start with medical schools and then residency, which is usually about five years long. And then you do a fellowship, which is a sort of a specialization after residency of one to two years. And eventually you get to work. And there are different types of path for radiologists. So the most, by far, most radiologists are what we call general community radiologists. And even though today here, we're gonna focus on the brain, generally a radiologist does essentially the whole body. So it involves knowing the anatomy of the entire body, the pathologies of the entire body. And this goes from the brain, the spine, the chest, the heart, the abdomen, the bones, the vessels. And there's a special field also for the breast from mammography. So it's a very broad specialty as a radiologist and it involves a lot of anatomical knowledge. Now, some radiologists will have a special interest when they work in the so-called community or non-academic setting. And so for example, someone with a neuro interest will have maybe more difficult neuro cases or will focus on neuro cases for one or two days a week. And then if we focus on what is an academic neuroradiologist is usually that entails working in an academic center where the radiologist will only do sort of brain and spine and often head and neck, which is the eyes, the throat and the oral cavity. And depending on the radiologist, there's a so-called diagnostic path, but there's also a lot of procedures, which is most commonly a lumbar puncture, getting CSF fluid from the back, but there's also about sort of repairing a vertebrae with vertebroplasty. And there's also a field for interventional vascular procedure that's being done. And the day-to-day practice, which might be obscure for many people, even within the medical field, is first that there's a large work, which is trying to design a protocol of all the sequence that will be acquired to answer a specific question. So for example, if a specific question is look for a tumor versus look for a brain aneurysm, this entails different images or sequences. And so a large part of the radiology work is actually protocoling or deciding which kind of images should be acquired to answer the question. And then the other large component is what we call reading or looking at the images, providing a report that will then try an attempt at a diagnosis and management if needed. And being an academic center is also a large part which is about teaching. So about residents and fellows and medical students. So to work in an academic center, you have to like teaching as well. Now, in terms of this, and when we say interventional neuroradiologist, most people will refer to this as the vascular field. So for example, if a patient has an aneurysm that has bled, then their interventional neuro-ideologist will go through the groin, all the way up with the arteries to the aneurysm, put metal coils in it to exclude it from the circulation and sort of repair it, if you will. Or they can go through the same way and remove clots in acute strokes to try and preserve the brain. Now, this is what I guess in my mind, I would call wet interventionalist, but there's also what I've been trying to call a dry interventionalist, which in the case of brain stimulation and functional neurosurgery, would refer to designing the sequence that are necessary to plan the surgery as best as possible, how to do special imaging post-processing that would be useful for the surgery or the procedure, and also how to generate a report that is specialized and really targeted towards the intervention to be as useful as possible and to provide as much value as possible for the neurosurgery and neurology team. So when we talk about functional neurosurgery, simplistically speaking, there are four stages that are relevant to clinical care. There's the patient selection, so which patient should undergo this type of procedure that we expect will respond best. And there's how to plan the surgery as best as possible such that we will increase the chances of success and minimize the chances of complication? And how can we monitor intra-op that the treatment is doing as it's intended to? And how can we monitor patients after the surgery to maximize the best outcome possible? So just a disclaimer before the work that I will be showing here, this is obviously not all my work. This is mostly done in collaboration and under supervision of Dr. Lozano and Fasano and in collaboration with Dr. German and Gavin Elias and many others within the Toronto team. So if we talk about patient selection, this is an example of a work that we did previously looking at the brain shapes of the brain volume of DBS patients that were to undergo DBS for depression. So we asked the questions, are there brain areas which are different in volume or shapes prior to the surgery that would help predict or help identify patients that will respond better to the procedure or not. And this is what Gavin found here in this paper where you can see the color blobs here identify regions which were different in the responder versus non-responder of depression DVS. And if we then did a sort of a more refined statistical analysis with a prediction component, this actually predicted who was gonna respond or not. So these regions were highly relevant. And so in the near future, you could think that looking at those areas would help the psychiatrist in addition to the clinical picture to select patients that shouldn't undergo DBS for depression. Now, in terms of surgical planning, these are some works that we've been doing in Toronto where for example, on the left, we looked at all the patients that had MR guided focus ultrasound, which creates a small thermal lesion or thalamus usually for tremor. And then if you looked at all the lesions that were done and you looked at where is the maximal area of efficacy, which is highlighted in the green region here, then you can then use that from now on in the next treatment to plan the surgery and make sure that the lesion corresponds exactly to this area and not spill over that would then cause potentially side effects. And similarly, we explored this avenue with DBS patient and a subthalamic nucleus for Parkinson's disease. And then we looked at whether the main cardinal features of Parkinson were to respond to different stimulation structure around the subthalamic nucleus. So we're hoping that by doing this, we can in the future, for example, stir the electricity or direct electricity preferentially towards these green spots, depending on the most disabling symptoms. Now, in terms of intraoperative monitoring, this is an example that we've, that Yergin did in collaboration, Yergin and Clements did in collaboration with the SickKids Hospital in Toronto, which is the pediatric hospital, where first they made a hypothalamic atlas. And then this was subsequently used to see whether a certain tumor in the hypothalamus in the pediatric population were to be most or less associated with seizure freedom after the treatment. And in this case, treatment was a laser interstitial ablation. Now the third role, which is how to monitor postoperative treatments. So it's an example that one of the other radiology fellow did about two years ago with us, where we looked at the MR guided focus ultrasound lesion in a thalamus and their appearances on the different sequences and how they supposed to evolve over time. So this is an example of what the lesion should be, should look like over time and on the different sequences. And so here the clinical implication is that if the lesion does not evolve in this direction or looks different, then that's probably because there's something that did not go exactly right with the treatment. And for example, we know if the lesion totally disappears by one year, for example, there's more high risk of the tremor recurrence. So this is how, for example, a radiologist here could add value by helping monitor the treatment, radiological appearances. And finally, another application that we've been working on is the DBS. One important step is after the surgery, trying to tailor the electricity for each patient. And this is usually done with several visits at the neurologist's office based on clinical feedback. And here, the concept that we've been trying to explore is whether the patterns of color of fMRI that we see when the DBS is being turned on in the scanner, whether this has any relationship with efficacy. So this is an example of two patients in A and B where in A you can see the row highlighted in green demonstrate a certain pattern that is lost when other contacts along the DBS leads are used. And again, when the optimal voltage is being used in the middle row, we can see that when the voltage become sub therapeutic in the upper row of B that the pattern is preserved but it's less statistical magnitude of efficacy. And when the voltage becomes supra-therapeutic, there's all the same patterns of colors maintained, but there's a lot of spillover in other areas. So this suggested to us that the patterns of color that we wanna see are highlighted in green. And if you change the contacts away from the optimal one or away from the optimal voltage, this pattern is lost. And so here we've been trying to use this fMRI tool as a tool, as an objective and rapid sort of marker of success for DBS patients. In this case, this was Parkinson. And then finally, another role that the radiologist can play in functional neurosurgery, because DVS has such a prominent role, is how to safely scan these patients. So we often, in this field of research, we all assume that MRIs is, you know, is an easy thing to get with these patients, often because it involves preoperative imaging. But there are strict rules that are enforced when scanning these patients with the electrodes inside the head after the surgery. And so it's an example how here we created phantoms that would replicate the DBS patients and sort of measure the temperature along the lead to make sure it wasn't heating up too much during the scanning as this is the main risk when scanning these patients. And at the time there was a need sort of to establish a procedure on how to scan these patients. And so this was our algorithm that we defined here in Toronto, whether first looking at the brand and model, making sure we know exactly what hardware is in the patient. And then depending if this was a research scan or a clinical scan, and there would be sort of two algorithms being followed, essentially always weighing the pros and cons of the MRI for the patient. And there was, this led us to sort of solve a problem here where at the time with the older DBS model, there were a lot of patients that needed a spine MRI while having the DBS in their brain. And here there was a lot of issue of trying to do this. So with using this phantom and sort of establish a safety margin, we could then design a spine MRI, a protocol that would save for these patients, but it would also at the same time answer the clinical question, for example, whether there was a disc or degenerative changes in the spine that were contributing to the gait problem in Parkinson's disease. So hopefully here with all these example, I could convince you that even though the radiologist is not typically seen to be directly involved in functional neurosurgery, I think as a profession, we can bring a lot to the field of functional neurosurgery and brain modulation at the several stages from patient selection to surgery planning, intraoperative monitoring, and finally monitoring the treatment long after the procedure.
- Thank you very much, Alexandre. It was really interesting. So now we can give the thing to Eric and we will take the questions after. - All right, thank you. All right,
so my name is Eric Middlebrooks.
Again, I am a neuroradiologist at Mayo Clinic in Florida And a lot of what I'm gonna talk about kind of builds on what Alex shared. I think we have a very similar idea. I really like his idea of the dry neurointerventionalist
'cause I do think that that is an evolution and radiology is that I don't think we really can just have a model that we sit in a dark room, you know, signing reports. And so I, you know, I'm at the, in the control room with my neurosurgeons planning the DBS surgeries, you know, there to help them with pre and post-operative targeting. And so I think that that's kind of the future in our field is that as we kind of sub-specialize that there's a lot more involvement outside of just the classical film interpretation. And so, let's see. So I was asked to give some background on some lessons and experiences of my own. And I can't say that I have like any profound experiences like I was there for the first DBS surgery or anything cool like that. So I wasn't exactly sure what to talk about. One of my mentees though, they said that they really loved my take on like wellness and burnout and that I should share that. So I'm gonna give you my perspective of things I learned through my own experiences. And I have to warn you, it's probably a little harsher than some of the burnout training out there, but I'll give you my perspective on the topic and then a little more positive note of tips for kind of finding your own niche. And I think this is really pertinent to those coming from the clinical translational side, but I think it also applies to a lot of people outside of those kind of core disciplines in neurology and neurosurgery of how you kind of tie in. And so the key to wellness is really doing as many training modules as you can. These are the key. No, I'm just kidding. In seriousness, wellness is like a critical part of our livelihood. And I think there's a few points that are not always talked about when we talk about these burnout training and things like that. And personally, I feel like a lot of these wellness discussions and training are kind of victim blaming, like you're not resilient enough. You don't know how to multitask. We can't do yoga, a lot of these kinds of things. But I think one of the things not commonly discussed that leads to burnout is really our expectations. And the one thing I stress to my trainees is this, There's no, if you want to do big things, great things, there's no shortcut. It takes what it takes to accomplish these things. And the idea that, you know, you can kind of do half the work as someone else and achieve the same thing just isn't a reality for most of us. So to do these big things, it takes what it takes to achieve. And I think, you know, at least as it currently stands, I haven't seen many grant applications that have a section on your wellness as part of the scoring criteria. So I think this is a disappointing realization, but I think it creates some zen if you actually consider the next points. And the first is don't measure yourself by others measuring stick. People have different priorities. They may be focusing far more effort towards something that you are willing or able to do, or maybe sacrificing in things that you don't want to sacrifice. And so the first is just setting your own realistic expectations, and importantly, having a timeline for those expectations. You have to prioritize the things in your professional and personal life that are most meaningful to you and not try to match what everyone else is doing. And so I know we have a lot of computational people on here. So I would consider this from a mathematical sense that you can consider like an academic power is a set amount of work per unit time. And so we can achieve a high power with a lot of work in a short time, but others may choose to spread that work over much more time to reach the same result. So I think if you use this blueprint to judge success by your own metrics, And obviously, if you have any external required metrics that may be required by your job description, those are important too. But using your own metrics to determine your own success is really important. And one thing that I've recently come to realize is careers are typically very long. Having not yet hit 40, sometimes I think, now what? What do I do? I'm not smart enough to keep thinking of new things for another 30 years. But really, I think most of us try to cram like 40 years of a career into about 10 years, and it's really not necessary. If you enjoy spending 85% of your waking hours doing science, then you may have career goals on a more expedited timeline, but not everyone feels that way. If you have other priorities or responsibilities, then maybe you need to consider hitting these professional milestones on a more extended timeline. But ultimately, I think the most important factor in wellness is to find your passion. And it turns out, we can tolerate a lot more work when it's something we enjoy versus something we hate doing. And to somewhat steal from Einstein here, a tedious task like writing a clinic note can make a minute seem like an hour, but doing a new MRI experiment or something data analysis that you really enjoy can make an hour seem like a minute. So this is academic relativity, right? So find your passion. So how did I find my passion? My story kind of begins during residency and fellowship at the University of Florida. And I think Alex did a really good job of kind of explaining the environment that is in radiology training. You know, we do not only neuro, but the whole body. So, you know, there's a pretty broad focus and then you start to narrow that down over time. And within this, my interest obviously was in neuro and I had worked some in this kind of growing field of functional neuroimaging, which at that time in the clinical setting was most exclusively applied to preoperative mapping for like epilepsy surgery or awake tumor surgery. And so I spent a lot of time on like MR physics, sequence optimization, paradigm design. There were things I really enjoyed and exploring brain function and mapping. And it just so happened that I was involved in several multidisciplinary clinical conferences, one of which was a movement disorder and DBS conference. And at that time, the stereotactic coordinates and atlases still reign supreme. But I was fortunate to work with a great DBS surgeon who was a big advocate for direct targeting and that was Kelly Foote at the University of Florida. And discussing with him, he frequently would talk about the need to confidently and accurately see structures that they're targeting. And this really led me to the realization that imaging needed to evolve to help facilitate better outcomes in functional neurosurgery. And so this was a lot of my interest and still is in developing new ways to try to visualize targets with improved accuracy and confidence. But as I began to learn more about neuromodulation, it just so happened there was another revolution on the horizon, one that greatly overlapped my other interest of functional neuroimaging. And so over the years, this niche has really grown and grown, I think, a lot of the people in this very meeting have been pioneers in this work. And while I think a lot of the early work was focused on refining traditional indications like tremor and Parkinson's, these tools turned out to be perhaps even more powerful than other emerging indications. And so today I'm gonna focus on one of these neuroindications, epilepsy, and show how some of these approaches that have grown out of traditional DBS indications really led to an acceleration in improving epilepsy DBS outcomes in just a few short years. But before we do that, I'll just give my perspective on kind of what helped me develop my research area. And again, I think these points are really important for clinical investigators and trying to kind of juggle clinical duties and research at the same time. So I think what has been most important to me is team science. And I strongly believe we accelerate science working together, sharing expertise, identifying and solving problems together. And this can be done both locally at your own institution, but also across the community. And I think we're very fortunate that within this neuromodulation community that there are a lot of people who feel this way, a lot of approachable people who really are focused on advancing the science and are willing to work together, share ideas. And the next is to question the way things are done. So never accept status quo as the best alternative. And this is particularly true, as I've shown, when you have an outsider viewpoint. You may be the only person in the room that's not biased to the way things have always been done. So what may seem like a stupid question is may just be a revelation that nobody thought to question before, like indirect targeting. So don't be afraid to question how things are done. And this ties into the last point is, identify where your expertise can solve problems in other areas. perhaps solutions haven't been discovered because everyone else on the multidisciplinary team lacked your background to apply new approaches. So listen to others' challenges and barriers and think how your expertise could potentially help solve them. And so with that, I'll start the discussion about epilepsy and just as some very basic background for anybody not familiar with this evolution and targeting is more historically the Atlas-based stereotaxis So using like a coordinate based system reference to something like the ACPC line was kind of our reference standard. And then this was refined in the operating room with micro electrode recordings and macro stimulation. And as MRI evolved, we started seeing more usage of atlases work to the patient's brain. And while these can be useful, they don't directly account for variations in functional anatomy between patients. So with a growing interest in MRI developments have allowed us to better visualize these targets and lead to what we call direct targeting as opposed to this indirect method. But to do this, it was obvious radiology really needed to catch up. So how can radiology help? So I'm gonna, this is kind of a little bit of an outline of what I'm gonna talk about. The first part is how we improve this direct targeting by better visualizing structures of interest. And then how we can help with kind of a newer concept of network surgery. So focusing more on targeting brain networks and network stimulation rather than just purely focused on these local-based targeting approaches. So imaging optimization has come a long way in the past few years with the advancement in MR technology. Here we see, you know, just kind of a traditional 3T SWI image and that came from a DBS pre-op scan versus the one on the right where you can see how we much better see these deeper structures like the GPI, the STN. And so this has been an ongoing evolution for many years in traditional indications like tremor and Parkinson's, but there really wasn't a great standard for how we do epilepsy. So applying these approaches to epilepsy, we can see, first, there's a big need for this, right? So the background in epilepsy is the most recent approved indication for DBS. It was approved by the FDA in 2018. and the anterior nucleus of the thalamus has been the most common target. If you look at the outcome studies, the one-year response rate was about 43% in the initial trial, but this improved to about 70% at long-term follow-up. But these follow-up studies were pretty heavily biased. So I think this is an overestimation of patient outcomes. And it turns out that within this cohort, that the anterior nucleus was entirely missed in 8% of patients requiring re-operation. But the subsequent, the post-hoc analyses actually show that a larger percentage of these patients that were in the ant were not optimally placed. So it really shows that there's a big gap in how we effectively target the anterior nucleus. And the last point I'll mention of these studies that we'll come back to in a minute is that the ant DBS response was greater in patients who had temporal lobe seizures. So why do we need direct targeting? So this should be actually fairly intuitive to us when we think about what we know in epilepsy, and that is that there are broad brain morphometric changes in patients with epilepsy. As we see in these patients with temporal lobe epilepsy, with right and left temporal lobe epilepsy, that we have these thalamic shape changes that occur, particularly affecting the anterior and medial portions of the thalamus. And so this is going to affect our targeting when we think about comparing to kind of a normal atlas or control population. And we see that reflected in the data. If we look at the difference between direct indirect coordinates, the thalamic volume greatly impacts the discrepancy between those, particularly when we look at the Y and Z axis. So this highlights the deficit created when we just assumed that the stereotactic coordinates should be similar across patients. Direct targeting also impacts our outcomes. So in this meta-analysis, in the studies using indirect targeting, the response rate was less than 50%, but the studies that used direct targeting had a 64% responder rate. But importantly, only 38% of patients were targeted by direct methods. So this shows there's a big gap. And the methods in these studies highly varied too. There were various 2D and 3D sequences used, some having kind of poor tissue contrast, the 2D sequences somewhat limiting your spatial accuracy because of the resolution. So we saw a big need for innovation in how we target the anterior nucleus. So the first question is to understand what are are we actually targeting? And in the SANTE trial, the original regulatory trial, is very broadly defined as the anterior nucleus, which in the DBS scale is a pretty sizable structure. So from early on, our idea, even kind of predating the FDA approval when we were doing pre-approval implants, we thought of, how do we have precision circuit targeting? You know, targeting the inflow and outflow areas of the anterior nucleus. And so we had been targeting specifically at the junction of the mammolith laminate track in the anterior nucleus. And so the concept here, our hypothesis was that these track, the part of the papaz circuit extending from the mammillary body to the anterior nucleus is mediated through this mammolith laminate track. And then the outflow through the anterior pole of the anterior nucleus, there are some fibers through the anterior limb of the internal capsule, but then also there's preceptal fibers that connect to the anterior cingulate. And so our idea was that we would wanna target those areas where the inflow outflow tracks occur, where you have kind of greater coherence of these circuits. And this was shown in several studies, post hoc analyses by several people. I think Fred Schaefer had a really nice study on this as well showing that the kind of sweet spot of these patients was really along the anterior ant and the ant MMT junction. So how do we target that? This was the way that we had been using. And this sequence is called FGATR. You may be familiar with it from other applications. It's been used in other DBS targeting applications. And the concept here is this is a high resolution 3D scan that's based off of the MPRAGE sequence, our 3D T1 gradient sequence. And we apply an inversion pulse. And that pulse is time where we have the recovery of white matter reaches this null point. So it effectively creates a white matter nulled image. And so using that, we see these structures really well. We see the memelithalamic tract here highlighted by the green arrow, and we can see the anterior ventral nucleus here and where these intersect. So this is how we directly target as we can visualize this area, and we place our electrode around the junction between the MMT and ANT. And you can see that in looking at the distance from the active contact to the memelithalamic tract termination, that there's a significantly different proximity and responders being much closer to this target point than non-responders. There are other ways to do this. I show this example just because, so this was a T2 space at 7T, and this was a really nice example of the asymmetry between the anterior nucleus and these patients. So you can imagine using stereotactic coordinates is gonna be a big problem in this patient. So this just kind of highlights the variation we can see. So as I mentioned, the NDBS responders were typically temporal of epilepsy patients, and seizures involving the limbic network. So one of the obvious questions that comes from this is why would we target the same nucleus for all forms of epilepsy? And indeed, there's been interest in new targets. And one of those is the centromedian paraphysicular complex which has been a common target in treatment of Tourette syndrome. But there's increasing interest in using this for epilepsy, particularly patients who have peri-rolandic seizures, things like Lennox-Gastaut and generalized epilepsy. And there's not a great trial yet showing outcomes for this. Many smaller studies with a pooled responder rate of about 73%. And the thought is that CM is a very broadly connected nucleus, in particular, part of the sensor motor network with connectivity to primary motor and premotor areas.
So there have been a few ways proposed of targeting this. It turns out that the central median nucleus is not well seen on most traditional sequences, like MP-RAGE or F-GATER. And so there's some different techniques that had been used to try to better see this. One is the MP2-RAGE sequence, which was published by Aaron Warren. So we took a slightly different approach in that the MP2-RAGE contrast, a lot of the thalamic contrast is derived from the first inversion time within that two inversion time sequence. So we tried to figure out how we optimize that to see the central median area. And so we had published this a while back as this edge sequence. And the concept of this is very similar to the F-gator, where we have the inversion time for the F-gator here where the white matter signal is nulled, with the edge is shifted further where we have the white matter recovery and gray matter recovery have a similar magnitude, but opposite polarity. And so what happens is voxels that have combined myelinated and non-myelinated tissue produces variable signal cancellation. And this effectively creates kind of a myelin weighted image. And so you can see here, The central median nucleus is somewhat difficult to resolve on traditional MP-RAGE and F-gater sequences, but on this edge sequence, we can see much better the boundaries for the central median nucleus. And at least early on, when we first came up with this, to get adequate SNR, you can see the scan times were fairly long. So this sequence is inherently a low SNR sequence because of the signal nulling effect. And so you can see here, admittedly, we can do this much faster with a little bit better quality now with some new modifications, but still long scans to do this. And so theoretically, 7T has drastically improved SNR and contrast and can allow us to do this at higher resolution with better scan time. So we see that here doing 50% reduction in voxel volume, we can get-- at eight minutes, we can get even better contrast and better SNR to see the CM. So now I'm gonna switch gears and talk about the non-invasive approaches to mapping brain networks and how that applies to DBS. And so a lot of this was already discussed by Alex. He's done a lot of this stuff in his cohorts. I think this is a very promising area in studying DBS is through the use of fMRI. But the first question is, we need to know how ant-DBS works. And there's really were not great human models for this. And so we used a similar approach that's been applied in a lot of non-epilepsy DBS studies by looking at the estimated volume of tissue activation and using an Atlas-based connectivity, resting state connectivity, to compare responders and non-responders. And what we see is that the responders have, This is the responders greater than non-responders map. And we can see that the responders had greater connectivity between multiple nodes of the limbic and default mode networks. And so the question is, what does the default mode network have to do with epilepsy? And it turns out this has been extensively studied in epilepsy. And in general, what we see is, particularly in temporal lobe patients, is that they have a baseline decreased connectivity in the default mode network. And importantly, at the onset of seizures, there's a deactivation of the default mode network. And then at the termination of seizures, there's a coinciding reactivation of the default mode network. So it seems like the default mode network in some way is like a regulator of the seizure propagation. And so the theory is that AntDBS may increase the threshold for this default mode network deactivation and limit seizure propagation. So the next question would be, how do we measure this effect with DBS, particularly in a single subject level? And when we talk about DBS with fMRI, you can somewhat broadly divide this into studying the acute stimulation effects by using kind of a block design of cycling the DBS on and off and looking at the difference. You can also do a task of interest with and without DBS. So perhaps looking at a motor task under the effects of DBS or something like that. You can also look at resting state alterations. So this would be looking at the on and off phase, what are differences in resting state connectivity, or looking at long-term STEM effects. So looking at say a baseline study preoperatively versus a long-term post-op scan with DBS off after a washout period. So there's a lot of different ways that you can use these tools to try to study DBS. But what we're interested in is looking at the acute effect of the stimulation. And when we use high frequency stimulation which is kind of the, has been the standard for epilepsy DBS, we see that by altering the stimulation off and on in these 30 second blocks that, and we can measure this with a surface ECG electrode. And then looking at the activation patterns, we can see that the activation indeed is in the default mode in limbic networks at the group level which kind of coincides with what we saw in the connectivity studies. When you compare the two though, it's interesting that the areas of activation with the high frequency stimulation were also areas that were estimated to be connected using the resting state connectivity and VTAC model, but not all of the connected areas were activated by high frequency stimulation. So it just shows us that indeed areas that are predicted as connected to a region may not be affected depending on the specific parameters used in the stimulation. Others have found similar results. So this is the Lozano group with Alex as well showing a similar pattern of activation with the anterior nucleus. And so I think this is a fairly reproducible and it's something that can pretty well be applied to single subjects, which is a bit of a challenge with resting state connectivity. So how might we use that? Here are examples of five patients who you can see had fairly similar stimulation volumes, again, targeting that anti-MMT junction. And what we see is that in these patients, one, three, and five look very much like the group level where we have these activation areas within the default mode and limbic networks in this very broad cortical deactivation. But when you look at these two patients, they have a very different pattern. And in particular, in some areas, you actually see the opposite, that we have this deactivation, such as like in the posterior cingulate cortex, very different look. And it turns out that these patients were the most difficult to achieve a good response in. So the question becomes why? And so we have electrophysiology data that I think helps shed some light on this. And in this study, We implanted patients with hippocampal electrodes and anterior nucleus electrodes, and we recorded from the hippocampus while we stimulated the anterior nucleus. And what you can see is that in some patients within the hippocampus, we're able to measure the potential, but in some patients we see nothing. And so these patients turned out to radiographically have mesial temporal sclerosis. And so this suggests to us that preexisting damage within these networks may limit the the propagation of the stimulation effect and therefore may limit the response that these patients may have to DBS. And so that's an interesting observation because theoretically this is something we may be able to predict preoperatively with fMRI to actually measure if patients have a favorable residual brain connectivity pattern that would allow them to respond to neuromodulation. So these effects have also been studied with CMPF-DBS in a swine model. And as predicted that the high-frequency stimulation does produce sensor motor network activation within the central median nucleus with a small amount of limbic and other areas, whereas the paraphysicular complex has a little bit of a different connectivity is that it's more limbic associated with some overlap with sensor motor and other networks. So this really supports the concept of this network specific targeting and epilepsy DBS. And it shows us that indeed by targeting these different areas and different parameters with our stimulation, we can indeed affect different networks. So the other thing that is valuable for using fMRI is that we can actually measure these in vivo effects of different stimulation settings. And so the frequency, pulse width, the stimulation location, the orientation of the E-field, all of these create a very complex model to simulate. And we also have devices that have greater complexity in programming options. So things like directional electrodes are using anodic versus cathodic stimulation, a lot of different things that are very difficult to model without having an in vivo biomarker. It also may serve as a clinical biomarker, and this is particularly helpful because we see increasing applications of DBS that don't have immediate clinical biomarkers that we see with some movement disorders, like with tremor, for instance, where we get a very rapid feedback on the response. So things like epilepsy, depression, dystonia, dementia, these are all things that do require quite a bit of time to optimize and can be, you know, a lot of work on the patient is trying to maximize this. So if we have imaging-based biomarkers, in theory may allow us to more accurately and rapidly optimize these patients. So directional electrodes are basically segmented electrode contacts that allow E-field generations and non-spherical distributions. And this allows greater customization, but it also adds to the complexity of programming. And it's more complex than just the E-field conforming to the structure or the nucleus. So I often see this mentioned as, you know, if you have the STN, it looks like this, can we make an E-field that matches this and we're set? And it turns out that to some degree that can be helpful, but it doesn't explain the whole picture. So can we use fMRI to try to model these things? So in this study, they looked at using directional stimulation. So the electrode was implanted and the stimulation was basically shifted around the electrode in four different positions and looking at the network effects. And so you can see that as the electrode position changes, the contacts change that it's cycling around the electrode that we do get different network effects. So this shows that that can be modeled looking at just the direction of the stimulation. But the other thing to consider is the E-field orientation. And so a lot of the effects of DBS are mediated via axonal stimulation and the stimulation intensity and effect varies with orientation of the E-field to the tracks and neurons. So stimulating these effects in vivo is quite challenging. And we can see that effect here where just by rotating the orientation of the E-field that we can measure very different responses electrophysiologically. And that can be shown again, using fMRI as a model. So this is in the same study, in this case, instead of changing the contacts rotating around them, the same contacts were used, but were stimulated in different models where we basically are rotating the E-field orientation around, and we can see that stimulating the same area, but changing the stimulation angle actually produces variable effects on motor, premotor and somatosensory cortex. So this again shows that even modeling the stimulation volume is only a snapshot of the picture that there are many other effects to consider, one of them being the direction of the E-field, but I also mentioned things like frequency. So for stimulation frequency effects, again, there's not a lot of in vivo data in humans that explore the effects of changing stimulation frequency and DBS, and this can have a profound impact on DBS effect, but it's not completely understood in many disorders. And so epilepsy is a great example. There's a rising interest in low frequency stimulation and a great debate about high frequency versus low frequency but it's still not quite understood what the difference is and who benefits from which. It's also important to remember the sensitivity of gray matter and white matter stimulation are frequency dependent. Low frequency stimulation is also more prone to have a suppressive effect, while high frequency more likely to produce activation effect. So we can use that to model the effects of stimulation in vivo. So again, looking at the acute stimulation effects between high frequency stimulation and low frequency stimulation. And so the concept is that high frequency, the high firing rate with shorter interpulse duration, it increases the mean spiking rates with neurons having less time to hyperpolarize, while low frequency has a long lasting hyperpolarization and it reduces the overall firing effect. So again, looking at anterior nucleus mapping, we can see that simply by changing the frequency that we get a very different effect. And so it remains to be completely understood what this means for a patient and who may benefit from which form of stimulation. We can measure the same thing in central median nucleus and paraphysicular nucleus. So again, the central median shows relatively similar effect on the sensor motor new network with high frequency and low frequency, which are shown the high frequency and low frequency. So you can see the stimulation effect is very similar despite if you use higher low frequency, but in the paraphysicular nucleus, there's more frequency dependent variation. So we see with high frequency, we get much more sensor motor activation that we don't see when we switch to low frequency stimulation. We also see there's a greater limbic effect with high-frequency stimulation. So the low-frequency stimulation, you know, theoretically you could say from this data that within the paraphysicular complex, given the more sensor and motor activation you see with lower frequency, you know, could this affect the outcomes in patients with periurelantic epilepsy. So here is just kind of similar data. You can see with the paraphysicular comparing high frequency on top to low frequency that the CM looks fairly similar from high to low, but the PF looks vastly different in different areas across the brain. So just to summarize, your connectomics is a powerful tool for measuring patient-specific in vivo effects of DBS, and it may serve as a biomarker to help guide device programming. fMRI is a marker of neurovascular response, but it's important to remember when we talk about these activation, deactivation, it can't easily determine the net effect of the of the neuronal activity. So for instance, excitation versus inhibition. So an activation of inhibitory neurons is a net inhibitory effect. So it's just important to remember that activation is not synonymous with excitation. And then we are looking to see future studies that integrate electrophysiology and more detailed clinical data can hopefully help us better understand the findings that we see on fMRI and may in helpfully integrating the electrophysiology clinical information and the imaging information. - Thank you very much, Eric. I think we can take questions now. We will start with the, I don't think we have questions. Yeah, we will start with the live questions and it's still time to ask questions in the question and answer channels if you want. You can maybe raise your hand.
Okay, so if there is no question for now, I can start with a question maybe. So you have talked about using fMRI to guide programming and I'm just wondering, oh, it could be integrated in clinical practice. What do you think about it? Because I feel like it's a lot of work to get functional fMRI for the patients and I expect that you need a lot of scanning time to like tell different contacts? Sure, I'll let Alex go. Yeah, sure I can. So yeah, I think as you explain, you know, the fMRI solution is not entirely perfect, far from that. But the first thing I think to also remember is, you know, when these patients do DBS surgery, it's not a small deal either, right? So these patients expect, the expectation of these patients is the postoperative care will not be simple, right? Like in the context of Parkinson's disease patient, the mobility is difficult. Going at the hospital for all these visits is not easy. And often the way the system is made now is they might travel from far away, right? For all these visits, because there's usually the expertise is in one big city. So the current approach is also not perfect. Now, when we started this, as you point out, you know, testing all the contacts, right, left, and, you know, it takes, it takes some time and some older patients don't like lying down in an MRI for that long. So that's what we are currently also working on is whether, you know, once we test one contact, for example, can we already know which, if this doesn't happen to be good, can we already predict which other ones should we be testing? So this would save a a lot of time. And so, so if we could predict the next setting, as opposed to do a trial and error that would save a lot of time, it seems we can do that. We're using a whole brain approach. And I think also in terms of costs, while fMRI is costly, especially in North American system, the clinical visits also, you know, not cheap over many years that these have to happen. And I think one sort of advantage of the fMRI that could happen is as now, you know, the new models are 3T eligible or compatible,
then this will remove technical barriers to doing this. And imagine if you're a patient that has to do, you know, a three hour drive to the hospital, you know, in a long, long distant future, if you could do the MRI and, you know, with the MRI technologist and maybe a nurse practitioner elsewhere, and then you could send the images, expertise center that might also be logistically helpful for these patients that come from far away. So yes, it's not a perfect solution, but as you know, with technological advances, I think we can make it better too. - Thank you. I think Andy raised his hand. I saw it and I will look for it. - Yeah. I just waited because there were also questions in the chat, but I can go first. So, so question to Alex as well, when you showed your, the fMRI activation maps from during DBS, there was this clear peak in the thalamus and that's, you know, not surprising, I guess it was STN simulation. Do you know which, which nucleus that was? Was it more VAM or VA or BL? - To be honest, this, this peak in the thalamus is a bit difficult because if you look at the older fMRI studies from the 2000, it was always the debate whether this is more of a direct, like how much of it is a direct propagation of the local stimulation versus an actual circuit stimulation I think is the first problem or question. Now exactly which nucleus? No, I don't think we looked, but it would be mostly ventrolateral part of the thalamus. So maybe more the motor component, roughly speaking with the eyes. - I think that- - Yeah, I meant like, you know, is it possible to just, to do some thing, but is it receiving with cerebellar, right? Because if it is in the, like, basically, I'm receiving VA or so, then you would, it is maybe a circuit effect. So it could be interesting to look into, but great work. Yeah. - I think we have a question from Freddie Schaefer. Like, so I will follow you to talk. - Hi.
Great, great talks, Alex and Eric, really beautiful. And thank you so much for these great talks. My question is for Dr. Middlebrooks. You showed an amazing, beautiful picture of all the difficult fiber pathways crossing the ENT target area for DBS and epilepsy. My question is kind of twofold. One, what is your best guess as to what is the fiber pathway responsible for therapeutic response? And two, are our normative connectomes or DTI in a patient good enough to visualize those? - Sure, so I think that, you know, targeting the memmolithic laminae tract is much easier due to its course within the thalamus. Stimulating, I don't think there's a lot of great data looking at stimulation closer to the anterior pole just because of where we typically position the electrodes. I don't know if other groups may have any data that could be used to look at that, but I think that's a very difficult target surgically. So I think we probably won't know. I think when you think about the constraints of surgical targeting, the memelothalamic tract is probably the easiest point. And so I think that it's kind of similar to what we talk about in like tremor, for instance. So this kind of zona inserta area as opposed to your subthalamic area where you have a greater bundle of the DRTT fibers coming in
given kind of a more compact surgical target, it's kind of probably a similar effect to the MMT. And so I think that that's probably what we're targeting. The fiber tracts, particularly the outflow, you know, the tracts through the septal area to the anterior cingulate, those are much more difficult to model. And so I think that from the connectome model, you have to be a little bit careful when using the normative connectomes. And I know that Andy and people have done a lot of work looking at the accuracy of patient-specific versus the models. But I think epilepsy, we have to be a little bit cautious because those patients tend to have far more anatomic variations in general than we see with like Parkinson's and things like that. So while there may be network abnormalities, the anatomic abnormalities I think are less common. I mean, I know you've seen some of these epilepsy patients are just wild how much they vary from normal. So I think we have to be cautious in the epilepsy realm when using normative connectomes. And then for, in the surgical planning realm, I think that the, it is very, it is difficult, particularly with deterministic methods to track the MMT through the, you know, the thalamus because of the FA with the gray matter, but it can be done. The, I think my perspective is that the F-Gator is really easy, right? You do it, you see the tracks really well. There's very little distortion, there's no post-processing. So I think that, yes, it could be done with tractography, but I think that it's a little bit more tedious solution than using the F-Gator. But when we talk about the kind of assessing the network effects and things like that, that data may be important in the long run as being able to look at not only the tractography, but also the connectivity, which I think in, again, in these epilepsy patients, there's such a range of variability from average. Your standard deviation is huge because of their disease. It's gonna be really important that we have patient-specific data. - Great, thank you so much. And I just wanna add, you have the record of showing the most beautiful slides of the thalamus in your talks. So thank you for that. Really, those 70 pictures are amazing. - Thanks, Fred. And good work. Fred is a-- he will not mention it, but he has driven a lot of the work around targeting and DBS and interior nucleus. So he's done really good work. Thanks, Eric. So I think we have a question for Kara Johnson. Yeah, I think we just have a couple of minutes left. But let's try to take this one. Tara, you're allowed to talk. - Okay. - Okay, hi. Great talks from both of you. Really enjoyed, especially all of the fMRI-DBS work. It's really exciting. My question is for Dr. Middlebrooks. You mentioned that in ANT-DBS, patients who had temporal lobe seizures seem to respond well. curious if you think that there might be some common network between ANT-DBS versus responsive neurostimulation in the temporal lobe, if that's something that you've looked at perhaps. And I guess a more simple question is how do they decide whether a patient with temporal lobe seizures would be implanted with ANT-DBS versus RNS? Yeah, good question. So RNS, the nice thing about RNS is the recording capabilities are really unmatched with DBS. The challenge though is even though it carries an FDA approval, a conditional scanning for RNS, it's difficult because the battery is a cranial implant, So it creates a huge amount of artifacts. So while theoretically they can be scanned, very limited what you can actually do. So we haven't really studied that group much. When it comes to who gets which, that's a great debate. I think it's very center dependent. There's not great head-to-head data. My personal thought is that chronic subthreshold stimulation is superior to responsive stimulation, but there's very little data that has suggested that may be the case, but nothing for sure. So I think it still is not fully settled who would benefit most. You're right that the population is very similar to who gets implanted. It just seems to be a little bit more experiential and center dependent. I think when we at our center, and I think others have kind of a similar idea is when RNS is particularly helpful in, the patients we implant most commonly are patients who tend to have poor lateralization of their seizure onset. So patients who have bitemporal onset or patients who seemingly have all their seizures coming from one side, but may have occasional events or something that are arising from the other side. So the idea is that by implanting them and monitoring them long-term and seeing these very long-term cyclical patterns and their seizures may give you a better idea of where their seizures are coming from. And we've seen some of these patients where like 95% of their seizures are actually only coming from one side. And so we actually will go back and ablate that side. So in some sense, I would say RNS is a little bit of a diagnostic tool as well. Whereas DBS, even though we do have things like sensing capabilities and all that, it really hasn't made much change in how we deal with epilepsy. So we have still a few questions in the chat, but I think, Alex, you don't have more time. You said you need to leave. Yeah, maybe five minutes and then I'll take it. Okay, so Patricia, can you go on with the question and answer? Yeah, so I can read one question, maybe from Calvin with one vote. I really like the demonstration of MTS potentially blocking the effect of stimulation based on those medial temporal electrodes. Do you have any thoughts on how similar blocking effects could be radiographically identified in other cohorts or in the absence of depth electrodes? I ask in the context of an Alzheimer's disease. - Sure, yeah, so it's a great question. So the data that is missing is the imaging correlate of that. So how we integrate electrophysiology
and the imaging side of it. Hypothetically, you may think that using like resting state connectivity that you may see impaired connectivity between these different nodes in the patients with MTS that may be what we're seeing electrophysiologically, but there's no data yet directly proving that that's the case. So I think that it remains more of a hypothetical that you could predict the network integrity through connectivity measures, imaging-based connectivity measures as a surrogate of that, but it's not proven. If there's anybody ... I don't know if there's been any animal studies that I'm not aware of, but no human data. - Thank
you. Would you have time for another question or? - Sure. - There is one by Luigi. What is your experience with the use of post-processing analysis on MRI sequence? Can they be easily integrated into routine surgical planning? - Sure, Alex, do you want to go first?
- Oh, no, I mean, to be honest, you probably have more experience than me with this whole clinical application, especially coming from the US, it might be a bit different story, but it seems that one of the main barrier to me, my limited experience is that first of all, the tool that have to be, you know, that can be used officially, you know, being recorded in a system of all that, I'll need the necessary approval. And the problem with these tools, I find often is it is non-flexible. And so we don't really know what happens in the background. And often, at least at this stage, the fMRI processing might have to be changed depending on the disease, the patient population. So until we, I guess, have a better or a more streamlined way of doing these kind of fMRI for DVS with the artifact and all this, it's a bit difficult, I think, to fully integrate it for all radiologists. I think for now it has to be done kind of offline with flexibility in the post-processing and sort of use as an adjunct of care until all the regulatory approval will, you know, can be obtained with this. - Yeah, it's the commercial side of advanced imaging seems to lag behind quite a bit. I would say probably 98% of people doing clinical tractography are still using like a single tensor model, which is like ancient technology, right? So it's largely driven by demand, right? If people, if the clinicians who are purchasing these packages and using these packages really demand that something else is done, then the companies don't have a lot of incentive to pursue that. Really at the end of the day, their best chance of selling something is if there's a billable indication tied to it. And since we don't yet have that as a billable indication for DBS, it's very hard to think about that being commercialized. But as Alex said, I think we're also still not quite settled. He and I actually had this discussion at the NIH not long ago. We still don't exactly know what's the ideal way of doing this in terms of ease of actually doing these kinds of tests, and then also with still getting reliable results. So I think there's still a lot left to be figured out about the best way to do this and the easiest way. It is not something that the, particularly the, measuring the stimulation effects under fMRI is a very tedious process on the acquisition side. And so I think we need help, you know, particularly from the manufacturers in coming up with better ways of being able to integrate the fMRI with the DBS platform. And so those, we've been discussing that. And I think as we've seen more literature and stuff coming out about the potential of this, they have started to maybe be a little bit more interested in how they can help us. And so we're hopeful that, you know, that relationship will continue to grow, but yet all of the advanced imaging stuff can be challenging both preoperatively and postoperatively to implement in routine clinical practice. - Thank you so much for answering questions. It looks like the time's up, but thank you so much for the talks. Andy had to leave, unfortunately, earlier, but he's saying bye and we are really grateful and thank you so much again and have a nice day. - Thank you. - Thank you. - Thank you everyone. - Bye. - Bye. Bye. [BLANK_AUDIO]